4. Alcohol
A number
of organic compounds that provide aromas in food are readily dissolved in ethanol but not
in water. You will invariably encounter dishes where alcohol is used for its chemical
properties, either as a medium to carry flavors or as a tool for making flavors in the
food available in sufficient quantity for your olfactory system to notice.
Note:
Ethanol can react with carboxylic acids in acid-catalyzed conditions, forming
compounds that then react with more ethanol to generate water and the ester compounds
that help carry aromas up into the nasal cavity.
Alcohol is often added to sauces or stews to aid in releasing aromatic compounds
“locked up” in the ingredients. Try adding red wine to a tomato sauce or dribbling a bit
of Pernod (anise liqueur) on top of a piece of pan-seared cod served with roasted fennel
and rice.
You can also make your own flavor-infused vodkas by adding diced fruit, berries,
herbs, or other spices to straight vodka. And since your concoction doesn’t have to be
shelf-stable like commercial varieties, you can generate better-tasting infusions. Don’t
limit yourself to just vodkas, either; try adding mint and a small quantity of sugar syrup
to bourbon whiskey and storing it in the freezer.
No, not entirely. Even though the boiling point of pure ethanol
(C2H5OH) is lower than that of water at
atmospheric pressure (173°F / 78°C), the intermolecular bonding between ethanol and
other compounds in the food is strong enough that its boiling point varies based on the
concentration of ethanol in the food and how the other chemicals in the food hold on to
it. The table to the right shows the percentage of alcohol remaining after various
cooking methods according to a paper published by researchers at the University of
Idaho.
Cooking method
|
% remaining
|
---|
Alcohol added to boiling liquid and removed from heat
|
85%
|
Alcohol flamed
|
75%
|
No heat, stored overnight
|
70%
|
Baked, 25 minutes, alcohol not stirred into mixture
|
45%
|
Baked/simmered, alcohol stirred into mixture:
| |
...for 15 minutes
|
40%
|
...for 30 minutes
|
35%
|
...for 1 hour
|
25%
|
...for 2 hours
|
10%
|
|
The term “fat washing”
comes from the process of using fat to “wash out” undesirable molecules, but it is
more useful in the home kitchen (and in molecular mixology) as a way of infusing
oil-soluble compounds into alcohol. If you use a non-neutral flavored fat—a fat that
has other molecules mixed in—some of the flavorful molecules will bind with the
alcohol molecules (it is a solvent, after all) and remain behind in the
drink. Why do this? Because you can create infused alcohols with flavors that
might not come out in traditional infusing. The flavors can either be native to the
fat (butter, bacon) or fat-soluble compounds bloomed in the fat before fat
washing. Create an infusion of 3–5% fat and 95–97% alcohol. Try 2 teaspoons (10g) of melted
butter with 1 cup (200g) of rum or 2 teaspoons (10g) of bacon fat (filtered!) with 1 cup
(200g) of bourbon. Let rest at room temperature for 12+ hours. Longer times and higher
temperatures will yield a stronger infusion, so you’ll want to experiment. Try using an immersion blender to kick-start the infusion. After infusing, place infusion in freezer until fats have solidified, and then
filter through a coffee filter or other ~20-micron filter .
Unfiltered.
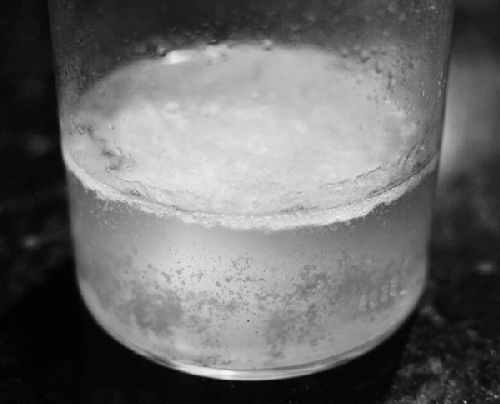
100 micron filter.
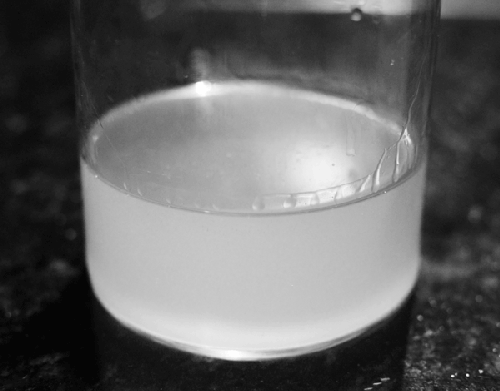
~10–20 micron filter.
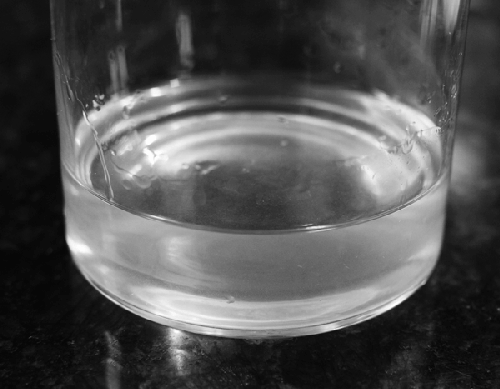
Notes Try this with blue cheese, nut butters, and other
fats. A key step in refining alcohol is the removal of undesirable
compounds. It’s impossible to remove every last “bad” molecule, but the more that
are removed, the better tasting the beverage will be. This is why “the good stuff”
costs more: refiners are able to remove more of the off-tasting compounds by
increasing the number of steps in processing or giving the alcohol longer to age,
which allows for better yield of the chemical reactions that remove the compounds.
Fat washing can be used as a DIY way to further refine an alcohol: the compounds
will bind with some of the fat molecules, which can then be removed by simple
filtration. Try using a neutral-flavored fat, such as lard, for refining without
altering the flavor.
|
In a small glass jar with a tight-fitting
lid, put: 1 vanilla bean, sliced open lengthwise and chopped into
strips to fit jar 1 oz (30g) vodka (use enough to cover vanilla
bean)
Screw lid on jar or place plastic wrap over top and store in a cool, dark place
(e.g., pantry) for at least a few days. Give the extract at least several weeks to
steep. Notes The vanilla bean can be left over from some other recipe. If you cook
with vanilla frequently, consider keeping the jar of vanilla constantly topped
off. Whenever you use a vanilla bean, add it to the jar, removing an old one when
space requires it. And as you use the extract, occasionally top off the jar with a
bit more vodka or other liquor such as rum. Play with other variations: instead of vodka, which is used for its
high ethanol content and general lack of flavor, you can use other spirits such as
rum, brandy, or a blend of these. The ethanol dissolves a number of compounds present in the vanilla
bean, including the compound vanillin, which gives vanilla its characteristic
flavor. Instead of vanilla beans, try using star anise, cloves, or cinnamon
sticks. Or try varying both solvent and substance (e.g., orange rind with Grand
Marnier). Flavored alcoholic drinks can be made with this same technique.
Instead of a large quantity of the solute (e.g., vanilla bean) and a minor amount
of solvent (e.g., vodka), place a small bit of the solute into a bottle of the
solvent. For an example, search online for nocino, an Italian
walnut liqueur made with unripe walnuts, aromatic spices, and
ethanol.
|
This is a simple cocktail and a darn good one. And having a simple,
darn-good cocktail in your repertoire can be handy. It only takes knowing one good
drink to impress that romantic potential. Put two or three sage leaves (fresh!) in a shaker and muddle with the back side of a
spoon. Add 1 part gin and 1 part pink grapefruit juice—say, 2 oz (50 ml) of each—and add
several ice cubes. Shake vigorously. Strain into a martini glass. Note |
Alcohol
isn’t the only solvent in the kitchen. The same chemical interactions that give alcohol
its magic apply to oil and water, which is why recipes call for steps such as toasting
caraway seeds in oil: the oil captures the molecules responsible for the characteristic
nutty flavors developed and released by heating the seeds.
But how does a solvent work? What happens when one molecule
bumps into another molecule? Will they form a bond (called an intermolecular
bond) or repel each other? It depends on a number of forces that stem from
differences in the electrical charges and charge distributions of the two
molecules.
Of the four types of bonds defined in chemistry, two are of culinary interest: polar
and nonpolar.
A molecule that has an uneven electrical field around it or that has an uneven
arrangement of electrons is polar. The simplest arrangement, where
two sides of a molecule have opposite electrical charges, is called a
dipole. Water is polar because the two hydrogen atoms attach
themselves to the oxygen atom such that the molecule as a whole has a negatively charged
side. When two polar molecules bump into each other, a strong bond forms between the
first molecule’s positive side and the second molecule’s negative side, just like when
two magnets are lined up. On the atomic level, the side of the first molecule that has a
negative charge is balancing out the side of the second molecule that has a positive
charge.
A water molecule is polar because the electrostatic field around the
molecule is asymmetric, due to the oxygen atom being more electronegative than the
hydrogen atoms and the resulting differences in how the two hydrogen atoms share
their electrons with the oxygen atom. (Electron sharing is another type of bond, a
covalent bond.)
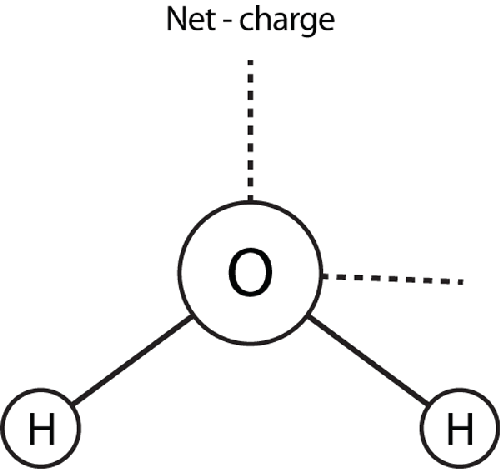
A molecule that has a spherically symmetric electrostatic field—that is, there is no
dipole, and the molecule doesn’t have a “side” that has a different electrical charge—is
nonpolar. Oil is nonpolar because of the shape in which the
carbon, oxygen, and hydrogen atoms arrange themselves.
In most cases, when a polar molecule bumps into a nonpolar molecule, the polar
molecule is unlikely to find an electron to balance out its electrical field. It’s a bit
like trying to stick a magnet to a piece of wood: the magnet and wood aren’t actively
repelled by each other, but they’re also not actually attracted. It’s the same for
polar-nonpolar interaction: the molecules might bounce into each other, but they won’t
stick and will end up drifting off and continuing to bounce around into other
molecules.
This is why oil and water do not mix. The water molecules are polar and form strong
intermolecular bonds with other polar molecules, which are able to balance out their
electrical charges. At an atomic level, the oil doesn’t provide a sufficiently strong
bonding opportunity for the negatively charged side of the water molecule.
Water and sugar (sucrose), however, get along fine. Sucrose is also polar, so the
electrical fields of the two molecules are able to line up to some degree. The strength
of the intermolecular bond depends on how well the two different compounds line up,
which is why some things dissolve together well while others only dissolve together to a
certain point.